The Application of Oxidants in Seawater
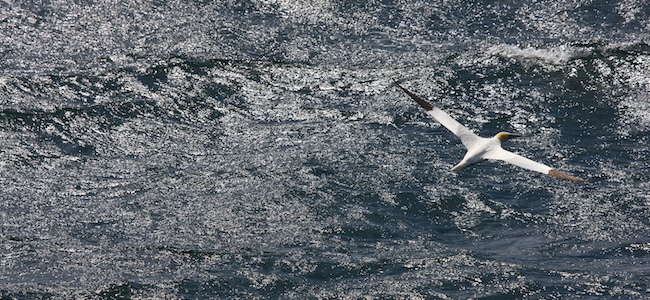
Seawater Disinfection
The disinfection of seawater is required for numerous applications, including desalination, ballast water, aquaculture, seawater pools/spas, and in cooling towers. Each application has specific considerations that are best met by a particular disinfectant, usually an oxidant. The oxidants include chlorine, bromine, chlorine dioxide, ozone, peracetic acid, hydrogen peroxide, ultraviolet light and advanced oxidation. In some cases, adding more than one oxidant, sometimes in a particular sequence, is most effective. To determine the most effective procedure for deactivating organisms of interest, empirical studies are required that vary the oxidiser, the concentration of the oxidant, and the contact time. Seawater presents a unique challenge for disinfection due to the production of unintended disinfection by-products (DBP).
In seawater, secondary oxidants are often produced that have different characteristics than the primary oxidant. Chlorine, ozone and peracetic acid oxidise the bromide ions in seawater to bromine, which is also an oxidant. If free ammonia is present in the seawater, chlorine and bromine will form chloramines and bromamines, which are also oxidants. Peracetic acid (PAA) maintains an equilibrium with hydrogen peroxide, which is also an oxidant. If more than one oxidant is present in seawater, the disinfecting capability will be a function of all of them. Since it can be difficult to distinguish the individual concentrations of oxidisers in the same seawater solution, a parameter called the Total Residual Oxidant (TRO) concentration is often used. However, this parameter can only give the approximate disinfecting power of a solution unless empirical studies are done. The TRO concentration is typically measured by a colourimetric method, oxidation reduction potential (ORP), or amperometry.
Total Residual Oxidant Measurement Methods
The most commonly used colourimetric method for measuring TRO is based on the colour-producing compound N,N-diethyl-p-phenylenediamine (DPD). The reagent contains DPD, potassium iodide and a neutral buffer. Oxidants either react directly with DPD or with iodide ions to form iodine which then reacts with DPD. The oxidant hydrogen peroxide also requires a molybdate catalyst to increase the reaction rate with iodide ions. The oxidised form of DPD, known as Wurster dye, is pink. However, DPD can be oxidised further to a colourless form (1).
Since there is no TRO standard available for calibration, the DPD test used to measure TRO is calibrated with standard chlorine solutions, and TRO is consequently often expressed in units of mg/l Cl2. DPD tests calibrated for other oxidants, such as bromine, ozone, peracetic acid, etc. may theoretically be converted to mg/l chlorine by multiplying the measured oxidant concentration by the following chlorine to oxidant molecular weight (MW) ratios. Calibrations generated for other oxidants may deviate from the molecular weight ratios.
MW Cl2/MW Br2 = 70.9/159.8 = 0.444 mg/l Cl2 per 1 mg/l Br2
MW Cl2/MW O3 = 70.9/48 = 1.477 mg/l Cl2 per 1 mg/l O3
MW Cl2/MW PAA = 70.9/76.05 = 0.932 mg/l Cl2 per 1 mg/l PAA
While DPD tests will accurately determine the TRO concentration of seawater treated with ozone, colourimetric ozone tests that employ the indigo trisulphonate chemistry should not be used to measure the TRO concentration of ozone-treated seawater. The reaction of indigo trisulphonate with the bromine formed in seawater as a result of ozone treatment is slow, and for commercial ozone tests, it is further inhibited by the addition of malonic acid (typically used to prevent chlorine interference).
In the ORP electrode method the voltage difference between a platinum electrode and a reference electrode is measured in a solution. The platinum electrode spontaneously gives up electrons to an oxidant in solution until a surface charge builds up that is equal to the ORP of the solution in units of millivolt (mV). The ORP of the solution is a function of the equilibrium redox reactions of the oxidants as described by the Nernst equation. The ORP response is logarithmic, so each 10-fold increase in the oxidant changes the ORP by -59.16 mV per electron transferred. For the hydrolysis of chlorine,
Cl2 + H2O ⇌ HClO + H+ + Cl-
two electrons are transferred; Cl2 (oxidation state 0) transfers one electron to HClO (oxidation state +1) and accepts one electron to Cl- (oxidation state -1). Therefore, the potential of the solution changes by -29.6 mV for each 10-fold change in Cl2 concentration. The ORP will be sensitive to the concentration change of any ions that are part of the redox reaction, for example H+ and Cl- for chlorine hydrolysis. However, in seawater the pH is buffered by carbonate, and the chloride concentration is large enough so that it is not sensitive to small variations. The reference ORP electrode is also a significant source of variability because the baseline ORP value with no oxidant added may vary significantly between sources of coastal seawater.
In the amperometric electrode method, a voltage difference is applied between two electrodes that is large enough to cause the oxidants in solution to be reduced at the surface of one of the electrodes. This causes a current to flow between the electrodes that is proportional to the total concentration of all the oxidants being reduced. The response is linear and therefore more sensitive than ORP. Also, the reference electrode is not a significant source of variability since the flow of current is zero without the addition of an oxidant regardless of the source of seawater.
TRO analysers are commercially available that use the colourimetric DPD method (Watts) or electrode methods (Thermo Scientific Orion). Also, test kits are available for measuring discrete samples by the colourimetric DPD method (CHEMetrics, Hach).
The CHEMetrics range of colourimetric test kits for the determination of residual oxidant in aqueous solutions use the industry standard DPD methodology, and patented CHEMetrics® Self-Filling Reagent Ampoule technology, delivering sensitivity and accuracy within two minutes. Premixed. Premeasured. Precise. Each kit contains 30 tests. The oxidant analyte reacts with DPD (N, N-diethyl-p-phenylenediamine) to form a pink product which can be measured visually or photometrically. CHEMetrics offers test kits for Bromine, Chlorine/Hypochlorite, Chlorine Dioxide, Hydrogen Peroxide, Ozone and Peracetic Acid.
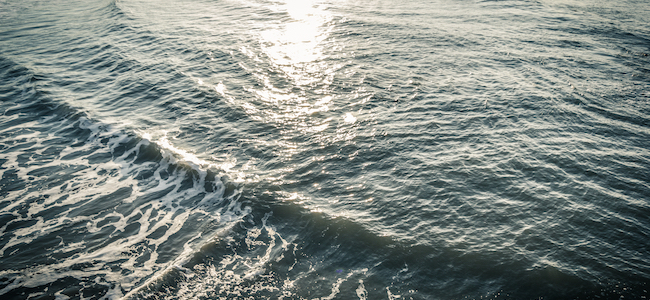
Oxidation Chemistry in Seawater
The characteristic of an oxidant that makes it a good disinfectant is its tendency to change the oxidation state of another chemical, a reductant, by accepting one or more electrons. This is called a redox reaction. In the oxidant, the oxidation state of an atom decreases and in the reductant it increases. The energy change from the oxidised state to the reduced state is the change in oxidation potential, which is in units of voltage since it involves the flow of electrons. The oxidation potential is a measure of the tendency of an oxidant to accept electrons. Generally, the larger the oxidation potential the stronger the oxidant.
Oxidant Potentials
The oxidation potentials of some oxidants (at standard conditions) commonly used for disinfection are given in Table 1 below.
Oxidant | Reduced Form | Oxidation Potential (V) |
---|---|---|
Hydroxyl Radical (OH*) | OH- | 2.8 |
Ozone (O3) | H2O | 2.07 |
Peracetic acid (CH3COOOH) | CH3COOH | 1.81 |
Hydrogen Peroxide (H2O2) | H2O | 1.78 |
Hypochlorous acid (HOCl) | Cl- | 1.48 |
Chlorine gas (Cl2) | Cl- | 1.36 |
Hypobromous acid (HOBr) | Br- | 1.33 |
Bromine gas (Br2) | Br- | 1.09 |
Hypoiodous acid (HOI) | I- | 0.99 |
Chlorine dioxide (ClO2) | ClO2- | 0.95 |
Iodine gas (I2) | I- | 0.54 |
If organic matter is present in seawater, the oxidant may produce harmful organic disinfection by-products (DBPs) some of which are regulated. If the DBP contains a halogen (chlorine, bromine or iodine) it is called a halo-DBP. While many hundreds of DBPs have been identified, those that are currently regulated in the USA include the trihalomethanes (THM), the haloacetic acids (HAA), bromate (BrO3–), chlorite (ClO2–) and in California and Massachusetts, N-nitrosodimethylamine (NDMA).
Maximum Contaminant Limits
The Maximum Contaminant Limits (MCL) for these DBPs issued by the US Environmental Protection Agency or state environmental protection agencies for municipal water are shown in Table 2 below.
The MCLs of DBPs are the same between England and Wales (2), Scotland (3) and Northern Ireland (4), and are based on the European Union (Drinking Water) Regulations 2014 (S.I. No. 122 of 2014 in the Irish Statute Book) (5). MCLs of DBPs are currently regulated in the USA (Stage 2 of the D/DBP Rule (2006)) (6), with the exception of NDMA which is regulated only in the State of California (7). The World Health Organization (WHO) values in the table below are achievable treatment performance values (8). In certain instances these vary slightly from the guideline values.
DBP | UK MCL | EU MCL | US MCL | WHO |
---|---|---|---|---|
Trihalomethanes (THM) | 100 µg/l | 100 µg/l | 80 µg/l | 60-300 µg/l |
Haloacetic Acids (HAA) | - | - | 60 µg/l | - |
Bromate | 10 µg/l | 10 µg/l | 10 µg/l | 10 µg/l |
Chlorite | - | - | 1,000 µg/l | 700 µg/l |
N-Nitrosodimethylamine (NDMA) | - | - | - | 0.005 µg/l |
Chlorine/Bromine
In fresh water where chloride is often present in significant concentration and bromide is in very low concentration, chlorine DBPs predominate, whereas in seawater where bromide is in significant concentration, bromine DBPs predominate. Bromine DBPs (and iodine DBPs) tend to be more cytotoxic and genotoxic than their chlorine DBP analogues (9).
Chlorine (Cl2) added to seawater hydrolyses to form hypochlorous acid,
Cl2 + H2O ⇌ HClO + H+ + Cl-,
which dissociates to form hypochlorite,
HClO ⇌ ClO- + H+.
The acid form is the stronger disinfectant. However, with a pKa of 7.53, the predominant form in seawater (pH 8.3) is ClO-. Despite hydrolysis, free chlorine concentration is generally expressed as mg/l Cl2 or ppm Cl2. The hydrolysed forms of chlorine react with the bromide ions, Br-, in seawater to form hypobromous acid, HBrO, and hypobromite, BrO-,
HClO/OCl + Br- ⇌ HBrO/BrO- + Cl-.
With a pKa of 8.65 HBrO is the predominant form in seawater. If free ammonia is present these oxidants form chloramine
NH3 + HClO ⇌ NH2Cl +H2O
and bromamine
NH3 + HBrO ⇌ NH2Br +H2O,
which are also oxidants. Furthermore, chlorite is formed by the reaction of two molecules of hypochlorite,
ClO- + ClO- ⇌ ClO2– + Cl-,
and chlorate is produced by the reaction of hypochlorite with chlorite.
ClO2– + ClO- ⇌ ClO3– + Cl-
Both chlorite and chlorate react with DPD (1). Analogous reactions produce bromite (BrO2–) and bromate (BrO3–). All the oxidants will contribute to the TRO concentration measured by the DPD method. The oxidants may react with natural organic matter in seawater to produce DBPs that include THMs, HAAs, halophenols and haloacetonitriles (10). These DPBs are not oxidants.
Chlorine Dioxide
The behavior of chlorine dioxide (ClO2) differs from other chlorine oxidants because it is a radical, having an unpaired valence electron. It is gas at room temperature that is very soluble in water but does not hydrolyse. It does not react with chloride or bromide ions to produce chlorine or bromine, nor does it produce organic DBPs or destroy organic matter. Chlorine dioxide disinfects by reacting with molecules containing amines like amino acids, RNA and proteins, and it can pass through cell walls as a dissolved gas (11). Residual chlorite may be introduced with chlorine dioxide because it is often used in chlorine dioxide generators (12).
2NaClO2 + Cl2 ⇌ 2ClO2 + 2NaCl
When chlorine dioxide is used in concert with ozone, chlorate is produced, which can accumulate in seawater to toxic levels (13). To avoid this, the ozone is applied before the chlorine dioxide.
Ozone
Ozone, O3, is an unstable gas that must be generated on-site. The powerful oxidising power of ozone can improve many water quality parameters besides disinfection, including the destruction of dissolved organic matter, controlling algae, colour, and odour. Secondary oxidants produced by the ozonation of seawater include chlorine, bromine, chlorate and bromate. Ozone reacts rapidly with bromide ions to form hypobromite,
O3 + Br- ⇌ O2 + BrO-,
which then may react with ozone to form bromate, leaving no residual ozone.
O3 + BrO- ⇌ 2O2 + BrO3–
Analogous reactions produce hypochlorite and chlorate. Although there is significantly more chloride (19,400 mg/l) than bromide (65 mg/l) in seawater, ozone reacts at a slower rate with chloride ions, producing about 83 times more hypobromite than hypochlorite unless all the bromide ions are consumed (14). Since it takes about 39 mg/l ozone to oxidise all the bromide ions in seawater, bromide is unlikely to be completely consumed for most disinfection applications. The reaction rate of ozone with bromide ions consumes all the ozone in about 10 minutes or less. In addition, another source of ozone consumption at the pH of seawater is the reaction with hydroxide ions, OH-, to produce ionised hydrogen peroxide and oxygen.
O3 + OH- ⇌ (ionised) HO2– +O2
Therefore, residual ozone will not usually contribute to the TRO concentration.
Peracetic Acid/Hydrogen Peroxide
Peracetic acid is produced by the reaction between hydrogen peroxide and acetic acid, and maintains an equilibrium with them.
H2O2 + CH3COOH ⇌ CH3COOOH + H2O
In seawater, peracetic acid produces the secondary oxidants chlorine and bromine, which may produce toxic DBPs. However, if the concentration of hydrogen peroxide is large enough it will reduce these secondary oxidants back to chloride and bromide ions, preventing or mitigating the formation of the DBPs (15).
Hydrogen Peroxide
A few parts per billion hydrogen peroxide occurs naturally in seawater from the photooxidation of organic matter (16). It degrades biotically due to an enzyme catalyzed reaction, and abiotically by a disproportionation reaction with itself, with transition metals, organic matter, free radicals and light. Although hydrogen peroxide has a larger oxidation potential than chlorine and bromine (Table 2) it reduces them to Cl- and Br-. It is often used as a dechlorination agent. Hydrogen peroxide is not known to react with bromide and chloride ions to form bromine and chlorine.
Ultraviolet Radiation
Ultraviolet radiation (UV) is light with wavelengths in the range of 100-400nm. It is divided into UVA (315-400nm), UVB (280-315nm) and UVC (100-280nm). Light in the UVC range transfers energy that is comparable to an oxidation reaction. It is commonly used for disinfection by causing disruptive reactions with the DNA of microorganisms. It also mineralises organic matter by creating hydroxyl radicals (17).
Advanced Oxidation
Advanced oxidation processes (AOP) involve the production of hydroxyl radicals (OH*) by combining UV with hydrogen peroxide, titanium dioxide or ozone, or hydrogen peroxide and ozone (peroxone). Hydroxyl radicals are extremely unstable because one of the normally paired orbitals in the oxygen atom is missing an electron, and thus unpaired. To pair the orbital, it accepts an electron from another chemical, and is therefore an oxidant. It is capable of completely breaking down organic chemicals to their H2O and CO2 components (18).
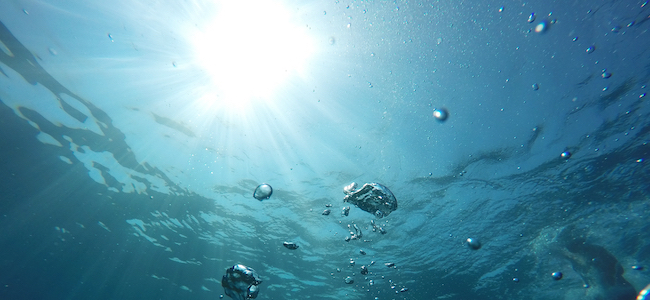
Applications
Desalination
Desalination is a major source of fresh water in the Middle East and a significant source in many coastal cities throughout the world. The two methods of desalination are thermal processes (evaporation and condensation) and reverse osmosis/ultrafiltration (RO/UF) through a semi-permeable membrane.
The desalination process begins with the pretreatment phase in which an oxidant is added to the seawater at the intake system to control biofouling. The water is then polished by coagulation and filtration, followed by destruction of the oxidant prior to desalination to protect the RO/UF membrane, if that is the method of desalination. During pretreatment, the important factors controlling DBP formation include the quality of the seawater and the choice of oxidant. While open ocean seawater is very uniform worldwide, coastal seawater may vary significantly in some components that may affect the formation of DBPs, such as organic content, inorganic nitrogen and bromide and iodide ions. After desalination there is a final disinfection step, in which an oxidant is added prior to discharge through the distribution system.
The degree of desalination is determined based on the application. According to the WHO, drinking water and irrigation water should be no greater than 450mg/l total dissolved solids (19). Often the desalinated water is mixed with other sources of fresh water to adjust the composition of the dissolved solids, particularly pH, hardness and alkalinity. If the added water contains significant amounts of organic matter, chloride, bromide or iodide ions, DBP formation may occur, depending on the choice of oxidant. The most commonly used oxidant for pretreatment and final disinfection is free chlorine, but other oxidants used include chlorine dioxide, chloramines and ozone (20). Chlorine dioxide has an advantage, especially in the pretreatment phase, because it will not oxidise bromide ions to bromine, avoiding the production of bromine DBPs, except in the presence of sunlight (21). However, it does introduce chlorite and chlorate, but by using extremely pure chlorine dioxide the concentrations of chlorite and chlorate can be kept below the WHO provisional guideline value of 700 µg/l (22, 23).
Ballast Water
Ships take up, transfer and discharge ballast water to maintain stability. It is usually composed of brackish to full strength seawater and contains any micro and macroscopic organisms that can pass through their filtration systems. Some of these species carried in ballast water may survive the journey if untreated, others may not. If the biological and physical conditions at the point of discharge are favourable, certain non-native species may establish themselves into these new environments to varying degrees and may cause serious ecological, public health and economic impacts. These ballast waster organisms must therefore be killed or inactivated below specified minimum limits to prevent them from infesting the locations where the ballast water is discharged (24). This is accomplished by a ballast water management system (BWMS).
The International Convention for the Control and Management of Ships Ballast Water and Sediments, developed by the International Maritime Organization (IMO) through the Marine Environment Protection Committee (MEPC), was adopted in 2004 to address this. The Ballast Water Management (BWM) Convention was adopted internationally in 2017 (24).
US waters are governed by the United States Coast Guard (USCG) Final Rule (2012), which is more rigorous than the IMO rules. Antarctic water also have their own special requirements (24). There are presently no international or US national regulatory limits for THMs or HAAs.
A BWMS is generally composed of a mechanism that either adds or electrolytically generates an oxidant based on the results of a DPD oxidant analyser or electrode oxidant analyser. The ballast water is dosed with enough oxidant to produce 1 to 15 mg/l TRO during transit (average 5 days), then neutralised to less than 0.1 mg/l TRO with sodium thiosulphate or sodium bisulphate before discharging. The required dose of TRO depends on the maximum allowable concentration of ‘active substances’, i.e., harmful organisms in the discharged ballast water (25). The oxidants include chlorine, chlorine dioxide, ozone, peracetic acid and hydrogen peroxide. Numerous commercial BWMS are available, e.g. Aqua Logistics Pte Ltd, Halogen Systems and Alfa-Laval.
Since the TRO content of ballast water tends to be measured periodically rather than continuously, it was found that the electrode measurement of TRO was less consistent than DPD measurement due to the equilibration requirements of the electrode with the sample (26). Besides chlorine and bromine, bromate and chlorate DBPs are also produced by both ozonation and electrolytic generation of chlorine in seawater. However, their formation can be reduced by adding hydrogen peroxide, which reacts with the chlorine and bromine converting them back to chloride and bromide (27). Increasingly sophisticated technologies are being applied to ballast water disinfection using advanced oxidation methods that generate hydroxyl radicals electrochemically (28) or with UV/hydrogen peroxide or UV/ozone (29).
Aquaculture/Aquaria
Recirculating mariculture systems (RMS) are closed loop aquatic animal production tanks that are in wide use for marine aquaria. In RMS systems that use artificial seawater the bromine and bromate DBPs produced by ozone can be substantially reduced by simply excluding bromide from the artificial seawater mixture (30). However, in real seawater bromate production may preclude the use of ozone as a disinfectant for systems containing sensitive organisms. Chlorine dioxide is not suitable for RMS because it results in an unacceptable amount of chlorate accumulation (31).
Recirculating aquacultural systems (RAS) in general employ filtration to remove faecal matter and prevent excessive ammonia levels, for instance, in order to allow water to be reused within the tank, and dramatically reduce the tank size and volume of water required to support fish or shellfish production. Particulates can cause problems for water integrity in RAS systems. Ozone is used in RAS as a disinfectant as well as to remove turbidity, algae and any taste, colour and odour. The effectiveness of ozone in deactivating various bacterial, fungal, protozoan and viral fish pathogens is dependent on concentration, exposure time, pathogen loads and also levels of organic matter, but higher ozone concentrations are a risk to fish causing gross tissue damage and associated mortalities as well as a risk to bacterial films on biofilters and ozone dosing concentrations require careful monitoring (32, 33).
Fish Processing
In the fish processing industry, hydrogen peroxide treated seawater was found to be more effective than chlorine or ozone at preventing biofilms in the distribution system because hydrogen peroxide could be used at much higher concentrations without adversely affecting the quality of life of the fish (34).
Saltwater Pool/Spa
Saltwater pools and spas contain about 1750-3500 mg/l sodium chloride. They have replaced most ocean pools, which were usually located on the coast and pumped seawater directly from the ocean. The disinfectant is produced by electrolytically converting chloride ions to chlorine.
Cooling Water
Cooling towers are used to dissipate waste heat into the environment. Investigators have compared the efficiency of different antifouling treatments for seawater cooling systems, including sodium hypochlorite, aliphatic amines, UV irradiation, chlorine dioxide and ozone (35, 36). While chlorine dioxide was the most effective biocidal oxidant at a low TRO dose of 0.1 mg/l ClO2, hypochlorite (35) and ozone (36) were more effective at higher doses. However, using chlorine dioxide to control biofouling in seawater cooling systems is promoted by water treatment manufacturers such as Scotmas Group over other oxidants due to its minimal DBP production (37).
References
- Harp, Danial L. (2002) Current technology of chlorine analysis for water and wastewater. Technical Information Series, Booklet No. 17. Hach Company, USA.
- Water Supply (Water Quality) Regulations for England and Wales (2016)
- The Public Water Supplies (Scotland) Regulations (2014)
- Water Supply (Water Quality) Regulations for Northern Ireland (2017)
- SI 122/2014 - EU Drinking Water Regulations (2014)
- USEPA's National Primary Drinking Water Regulations (NPDWRs)
- California State Resources Control Board. (2017) Groundwater Information Sheet: N-Nitrosodimethylamine. California State Resources Control Board. Retrieved 19 February 2022 from
https://www.waterboards.ca.gov/gama/docs/ndma.pdf - WHO's Guidelines for Drinking Water Quality (GDWQ) 4th ed. inc. 1st Add. (2017)
- Plewa et al. (2010) Mammalian cell cytotoxicity and genotoxicity of the haloacetic acids, a major class of drinking water disinfection by-products. Environmental and Molecular Mutagenesis, V51, 871-878.
- Adenekan et al. (2009) Transport and Fate of chlorinated By-Products Associated with Cooling Water Discharges, Proceedings of the 1st Annual Gas Processing Symposium.
- Lenntech B.V. Disinfectants: Chlorine Dioxide. Retrieved 22 January 2022 from
ttps://www.lenntech.com/processes/disinfection/chemical/disinfectants-chlorine-dioxide.htm - Gordon, G., and Tachiyashiki, S. (1991) Kinetics and mechanism of formation of chlorite ion from the hypochlous acid/chlorite ion reaction at pH 6-10. Environmental Science & Technology, V25, 468-474.
- Gordon et al. (1999) Ozone and chlorine dioxide: Similar chemistry and measurement issues. The Journal of the International Ozone Association, V21, 447-464.
- Jung et al. (2017) A kinetic study of ozone decay and bromine formation in saltwater ozonation: effect of O3 dose, salinity, pH and temperature. Chemical Engineering Journal, V312, 30-38.
- Shah et al. (2015) Peracetic acid oxidation of saline waters in the absence and presence of H2O2: Secondary oxidant and disinfection by-product formation. Environmental Science & Technology, V49, 1698-1705.
- Moffett, J.W., and Zafiriou, O.C. (1990) An investigation of hydrogen peroxide chemistry in surface waters of Vineyard Sound with H218O2 and 18O2, Limnology and Oceanography, V35, 1221-1229.
- Penru et al. (2012) Application of UV and UV/H2O2 to seawater: Disinfection and natural organic matter removal. Journal of photochemistry and photobiology A: Chemistry, V233, 40-45.
- Negarash et al. (2018) Potential for Formation of Disinfection By-Products from Advanced Oxidation Processes. Report DWI 12852.02.
- World Health Organisation. (2003) Total dissolved solids in Drinking-water. World Health Organisation, Geneva.
https://www.who.int/water_sanitation_health/dwq/chemicals/tds.pdf - Kim et al. (2015) Disinfection by-product formation during seawater desalination: A review. Water Research, V81, 343-355.
- Danesi et al. (2007) Chlorine dioxide disinfection technology to avoid bromate formation in desalinated seawater in potable waterworks Desalination, V203, 312-318.
- Palacios et al. (2015) Chlorine Dioxide as Disinfectant for Pretreatment in Seawater Desalination Plants. AWWA/AMTA Conference in Orlando FL.
- WHO. (2005) Chlorate and Chlorate in Drinking-water. WHO/SDE/WSH/05.08/86
- UK Maritime and Coastguard Agency. Control and management of ballast water. Retrieved 22 January 2022 from
https://www.gov.uk/guidance/control-and-management-of-ballast-water - Kim et al. (2016) Consideration on the maximum allowable dosage of active substances produced by ballast water management system using electrolysis. International Journal of e-Navigation and Maritime Economy, V4, 88-96.
- Zimmer-Faust et al. (2014) Evaluation of approaches to quantify total residual oxidants in ballast water management systems employing chlorine for disinfection. Water Science and Technology, V10, 5-93.
- Jung et al. (2014) Formation of bromate and chlorate during ozonation and electrolysis in seawater for ballast water treatment. Journal of the International Ozone Association, V36(6)
- Moreno-Andrés et al. (2018) Inactivation of marine heterotrophic bacteria in ballast water by an electrochemical advanced oxidation process. Water Research, V140, 377-386.
- Moreno-Andrés et al. (2017) UV-based technologies for marine water disinfection and the application to ballast water: Does salinity interfere with disinfection processes? Science of the Total Environment, V581-2, 144-152.
- Qiang et al. (2015) Formation of disinfection by-products in a recirculating mariculture system: Emerging concerns. Environmental Science: Processes & impacts, V17, 471-477.
- Wang et al. (2018) Characterization of Brominated Disinfection Byproducts Formed During the Chlorination of Aquaculture Seawater. Environmental Science & Technology V52, 5662-5670.
- Gonçalves, C., and Gagnon, G. (2011) Ozone application in recirculating aquaculture system: An overview. Ozone Science and Engineering, V33(5), 345-367.
- Summerfelt et al. (1997) Review of ozone processes and applications as an oxidising agent in aquaculture The Progressive Fish-Culturist, V59, 94-105.
- Shikongo-Nambabi et al. (2010) Evaluation of oxidising disinfectants to control Vibrio biofilms in treated seawater used for fish processing. Water SA, vol.36, n.3
- López-Galindo, C., Casanueva, J.F. and Nebot-Sanz, E. (2010) Efficacy of different antifouling treatments for seawater cooling systems. The Journal for Bioadhesion and Biofilm Research, V26(8), 923-930.
- Al-Bloushi et al. (2018) Performance assessment of oxidants as a biocide for biofouling control in industrial seawater cooling towers. Journal of Industrial and Engineering Chemistry, V59, 127-133.
- Scotmas Group. Is ClO2 Safe for the Environment? Retrieved 22 January 2022 from
https://www.scotmas.com/chlorine-dioxide/is-clo2-safe-for-the-environment.aspx